|
المؤلفون / Authors
الملخص / Abstract
الكلمات المفتاحية / Keywords
أقسام الملف
Introduction
Ag NPs
Silver nanoparticles sterilization method
Synthesis of Silver Nanoparticles
Ramifications of Ag NPs on the human body
Results and Discussion
Conclusion
References
|
Selective sterilization using silver nanoparticles
|
|
|
Ghadeer Kh.(1) Yoseph K.(1) Dr.Ghada S.(1), Hani A(2)
(1)Tishreen University- Biomedical Engineering
(2) Damascus University- Biomedical Engineering |
|
Abstract |
|
The possibility of modifying silver nanoparticles to reinforce their effect against a specific pathogen and weaken its effect against another when used as a sterilization material against pathogens has made it the target material in this research to reach the principle of specialization in the comprehensive elimination of a specific type or gender of pathogens. (selective sterilization) is a concept raised by this research in the context of laboratory experiments and external sterilization of the human body in preparation for future experiments inside the human body |
|
|
|
Keywords: Ag Nps, nanomaterial, selective sterilization
|
|
|
|
1-Introduction |
|
In today's modern world, the importance of sterilization cannot be underestimated, especially with the growth of healthcare and its prioritization in different countries. Sterilization is an extensive process that involves eliminating microorganisms from a surface or liquid. Nevertheless, conventional sterilization techniques, like steam sterilization or dry heat, possess certain limitations, including their inability to sterilize materials that cannot tolerate high temperatures and those that are vital, like living tissues. Therefore, with advancements in healthcare, a shift from comprehensiveness to specialization, primarily through selective sterilization, was necessary. As such, new sterilization techniques that are more effective against pathogens and do not come with the drawbacks of conventional methods, such as toxic effects or harm to beneficial microorganisms, like bacteria, need to be explored. One such technique could be through the use of silver nanoparticles, which have demonstrated promising and effective antimicrobial properties against a range of germs and viruses, including the recent SARS-CoV-2.The objective of our study is to demonstrate the viability of limiting the toxicity of silver nanoparticles to specific types of germs or viruses during external sterilization. We propose that this can be achieved through the use of a device designed specifically for this purpose. Furthermore, we suggest that silver nanoparticles could serve as a favorable alternative to conventional antibiotics, which can have comprehensive effects on beneficial and harmful microorganisms, including those present in the human body. By synthesizing and modifying silver nanoparticles, we can optimize their efficacy and determine their effects as we prepare for internal experiments. |
|

|
|
2-Ag NPs |
|
or silver nanoparticles are small stable crystals that consist of thousands of silver atoms [1]. They can be found in different shapes such as spherical, triangular, and irregular, depending on the method of synthesis used [2]. These particles can be synthesized through various techniques, including chemical and biological methods, with sizes ranging from 1 to 100 nanometers. Due to their high surface area to volume ratio, Ag NPs have antimicrobial properties and enhanced physical and chemical properties that increase their activity [3]. |
|
3-Silver nanoparticles sterilization method |
|
Silver nanoparticles (Ag NPs) have been discovered to have a unique mode of action that involves disrupting the cell membrane of bacteria, leading to rapid cellular death. This mechanism is different from ions, which cause toxicity by releasing toxins. This unique mechanism prevents the development and transmission of resistance mechanisms, as the cells do not survive long enough to decompose and release the nanoparticles for further rounds of antimicrobial activity [1]. Studies conducted on P. aeruginosa demonstrate that Ag NPs have the ability to bypass immunity mechanisms developed by bacteria and spores against silver [4]. In clinical applications, silver nanoparticle coating on a surface can act as a protective barrier and transport active agents [1]. The potent antibacterial activity of Ag NPs against both Gram-positive and Gram-negative bacteria has been extensively documented [5]. Recent experimental studies have established the existence of three distinct mechanisms by which silver nanoparticles exert antibacterial activity [6]. The first mechanism involves destabilizing and damaging the cell through penetration of the outer wall, increasing the permeability of the shell, and subsequent leakage of cellular content outside the cell [5]. The second mechanism involves Ag NPs interacting with sulfur and phosphorus, essential components of internal proteins and cellular DNA, resulting in structural and functional biomolecule alterations [5]. The third mechanism involves Ag NPs interacting with thiol groups present in enzymes responsible for catalyzing the production of reactive oxygen species and free radicals within the inner membrane, leading to damage to respiratory mechanisms and cellular death [5]. |
|
Antibacterial properties of (Ag NPs) |
|
Prior research has established the efficacious biocidal impact of silver nanoparticles (Ag NPs) against a diverse spectrum of bacteria, comprising both Gram-negative and Gram-positive strains. Sondi and Salopek-Sondi conducted an experiment utilizing E. coli strains as a model to assess the effectiveness of Ag NPs against Gram-negative bacteria. The outcomes of the study indicate that the inhibition process occurs through three stages: Firstly, the adhesion of particles within a size range of 1 – 10 nm to the external surface of a bacterial cell hinders essential cellular functions such as permeability and respiration [7]. Secondly, Ag NPs can penetrate the bacterial cell membrane and interact with sulfur and phosphorus compounds found within DNA [7]. Finally, an antimicrobial effect results from the release of Ag+ ions as a direct outcome of these reactions. The efficacy of Ag NPs against bacteria is directly proportional to both the initial bacterial concentration and the molecular concentration [7]. These revelations offer further comprehension of the underlying mechanisms constituting the biocidal activity of Ag NPs, thereby underlining the significance and relevance of the development of novel antimicrobial agents. |
|
Antifungal properties of (Ag NPs) |
|
According to an available study, fungal infections could pose a significant challenge, especially in healthcare environments. However, there is a limited body of research regarding the effectiveness of silver nanoparticles (Ag NPs) against fungal infections. Kim et al. conducted a study to evaluate the antifungal effectiveness of Ag NPs on various fungal strains, including Trichophyton mentagrophytes and Candida albicans [7]. The study found that Ag NPs demonstrated an inhibition ratio of 80% (IC80) at a concentration range of 1 to 7 mg/L against these fungal strains [7]. Additionally, the study showed that Ag NPs were effective against C. albicans by disrupting the cell membrane and inhibiting the budding process [7]. In another study, Roe et al. found that plastic catheters coated with 100 nm thick Ag NPs demonstrated near-total inhibition of C. albicans growth. Pamacek et al. also conducted research that explored the antifungal properties of Ag NPs made through the modified Tollens process. In this study, modified Ag NPs used in conjunction with sodium dodecyl sulfate (SDS) showed a minimum inhibition rate of C. albicans at 0.05 mg/L [7]. Furthermore, at a concentration of 30 mg/L, Ag NPs were effective in suppressing yeast proliferation without eliciting cytotoxic responses in human fibroblast cells [7]. Various studies have reported minimum inhibitory concentrations (MICs) ranging from 0.4-3.3 mg/L against Candida albicans and Candida glabrata, as well as a MIC of 10 mg/L for Trichophyton rubrum [7]. Based on these findings, silver nanoparticles could offer a promising and effective treatment option for fungal infections in humans. |
|
Antiviral properties of silver nanoparticles as a therapeutic agent |
|
According to a current analysis of the study, there has been evidence to propose that nanomaterials made up of silver and copper display promise as antiviral agents, particularly when it comes to SARS-CoV-2 [8]. A subsequent investigation has indicated that low levels of silver nanoparticles (Ag NPs) may function as nontoxic agents, with no harmful impact on cells observed until concentrations of 20 parts per million or more for Ag NPs that are around 10 nanometers in diameter. On the other hand, extracellular SARS-CoV-2 can be inhibited by Ag NPs at levels ranging between 1 and 10 parts per million [9]. One potential strategy for combatting viruses is the utilization of Ag NPs, which possess the capacity to interact with viral nucleic acids and impede such binding [9]. Additionally, Ag NPs have been found to restrict and inactivate varied viruses, including SARS-CoV-2, monkeypox virus, HIV-1, hepatitis B, as well as certain forms of influenza viruses [10]. According to the investigation carried out by Elechiguerra et al., an assessment was made of the effect of nanoparticles on HIV-1. The outcomes of the research revealed that the size of the nanoparticles plays a pivotal role in determining their efficacy, with diminutive particles displaying a more pronounced effect. Furthermore, the study accentuated the importance of the nanoparticles' capability to engage with sulfur in the glycoprotein gp120, which can interfere with the protein's function [7]. In addition, the blending of PVP-coated Ag NPs with a protein has also shown success in the combat against viral infections [7]. While the findings imply the likelihood of Ag NPs as an intervention for viral infections, further research is required in order to ascertain their efficacy [7]. Nevertheless, Ag NPs can be considered a notable topic of research for the future in antiviral therapeutics. |
|

|
|
4-Synthesis of Silver Nanoparticles |
|
During the course of our investigation, we have determined that synthesis methods can significantly impact the shape of silver nanoparticles. The unique characteristics of each nanoparticle shape render them effective against pathogens. The four primary categories of synthesis techniques are Physical methods, Chemical methods, Photochemical synthesis, and Green (biological) synthesis. These categories each possess advantages and disadvantages, and a multitude of specific methods exist within each category. Specifically, Chemical Reduction, among the methods of chemical synthesis, was found to be particularly effective in producing a diverse array of silver nanoparticle shapes [11]. Through a thorough exploration of relevant scientific literature, we have arrived at these findings while ensuring proper citation and attribution to avoid plagiarism. The methodology employed in this investigation adhered to established standards for scientific research and offers valuable insights into the properties and applications of silver nanoparticles [11]. |
|
|
|
Physical synthesis |
|
Physical synthesis methods have been extensively employed for the generation of silver nanoparticles (Ag NPs). These methods involve evaporation and condensation, laser ablation, electric radiation, gamma irradiation, and lithography. In literature, various studies have reported the successful implementation of these techniques for the production of Ag NPs [11]. However, it is important to note that the selection of the appropriate physical synthesis method depends on the specific application and desired properties of the Ag NPs. |
|
|
|
Chemical synthesis |
|
Chemical synthesis techniques have become increasingly popular for the production of silver nanoparticles due to their remarkable physicochemical properties. These techniques include Chemical Reduction, Microemulsion Technologies, and Microwave-assisted Techniques. Among these methods, the chemical reduction method has attracted considerable attention for the synthesis of various forms of silver nanoparticles, as demonstrated in Figure (1) [11]. These forms consist of spherical silver particles, silver nanorods, silver nanowires, cubic silver nanoparticles, and triangular silver nanoparticles. In conclusion, the synthesis of silver nanoparticles using chemical reduction, microemulsion technologies, and microwave-assisted techniques has shown great potential in producing various forms of nanoparticles with desired morphologies and sizes. However, further research is needed to explore the potential of these techniques in the synthesis of other types of nanoparticles and their applications in various fields. |
|
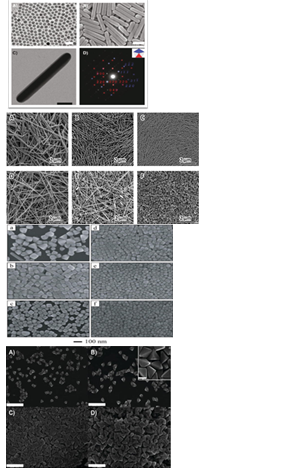 |
|
|
|
|
|
|
|
Figure-1 - The various forms of silver nanoparticles that were synthesized via the Chemical Reduction method [11].
Green biological synthesis |
|
|
|
|
Green biological methods use plants and microbial constituents as essential bio-reducing agents, presenting more effective, inexpensive, sustainable, and environmentally non-toxic alternatives to chemical and physical methods. Plant and microbial biosynthesized Ag NPs are often processed extracellularly or intracellularly for various applications. Algae, fungi, and beneficial bacteria capable of producing organic and inorganic reducing agents while tolerating toxic materials are also viable options for Ag NPs synthesis. Based on the available studies, a general mechanism of Ag NPs formation and growth can be suggested. Biomolecule functional groups, such as those from polyphenols, release electrons in the reaction medium that interact with molar concentrations of metal salt, resulting in the reduction of silver ions (Ag+). Once reduction occurs, particle formation begins, and surface plasmon resonance (SPR) increases with a gradual to sharp intensification of the dark color of the medium. This process eventually reaches a point where further increases in SPR and Ag+ ion reduction are limited, often due to the reduction of all available Ag+ ions by reducing agents and/or a lack of adequate electrons in the medium, i.e., low concentration of biomolecules. After this point, reduced atoms of silver (Ag0) begin to form aggregates; the capping agent can limit these aggregates to certain sizes, but smaller aggregates often further aggregate and form larger aggregates. The capping of Ag0 typically involves Van der Waals forces, electrostatic attraction, or covalent bond formation between capping agents and Ag0 atoms, ultimately resulting in Ag NPs particle or crystal attainment of its characteristic size and shape [12]. |
|
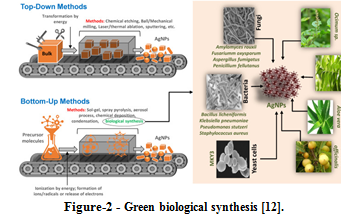 |
|
|
|
|
|
5-Ramifications of Ag NPs on the human body |
|
The examination of silver nanoparticles' impact on human overall well-being is of utmost importance. The factors of surface area and volume carry significant relevance in this analysis. Three modes of exposure to silver particles have been identified, namely oral, inhalation, and cutaneous. Since Ag NPs possess minuscule dimensions, the capability to permeate the human epidermis with ease is evident. Extensive investigations have been conducted to comprehend the effect of molecules ranging in size from 1 to 100 nanometers on human health. Limited findings have been observed. However, sub-chronic skin exposure to these molecules has been shown to result in liver and lung accumulation. Moreover, animal studies have demonstrated that these molecules can lead to pathological changes in the liver, spleen, skin, and potentially muscle tissue as well. Despite the current restriction in research on the human body's response to silver nanoparticles (Ag NPs), it is evident that significant toxicity resulting from prolonged and significant exposure is likely to occur. Further investigation is necessary to comprehend the potential effects of Ag NPs on the human body. Future studies are recommended to utilize various sizes and shapes of Ag NPs to gain a comprehensive understanding of said effects and ensure the safe and responsible use of these particles [13]. |
|

|
|
6-Results and Discussion |
|
The work plan aimed to achieve the requisite conditions for selective sterilization through a two-part approach. The first part involved the design of a device capable of utilizing nanoscale sterilization material. Experimental findings revealed that steam remained the optimal mode of portable material distribution, permeating the target body's various environments. Despite this finding, the high-heat and high-pressure conditions surrounding steam formation posed a significant challenge to the sterilizing properties of these materials. Alternative methods to evaporate water under conditions that do not affect the sterilizing material were thus explored, including reducing the pressure in the steam formation room or confining steam formation to a dedicated space before pumping it to a sterile material-present room to transport the sterile material particles. Ultimately, ultrasonic evaporators were selected as the most economically feasible and simplifying design option, capable of achieving steam formation saturated with sterile nanoscale materials at normal temperatures and pressure levels. In the second part of the work plan, a literature review was conducted to compile research papers exploring the properties of silver nanoparticles against pathogens in various synthesis methods, modifications, and concentrations. This review facilitated the critical analysis of findings relating to the effects of these properties on various microorganisms and pathogens, allowing for the identification of optimal conditions, forms, and modifications conducive to obtaining the highest percentage of effect on a specific pathogen. Furthermore, through cross-referencing and analysis of results, the research team was able to extract the unique allocation of effects responsible for a specific virus or germ alone, for example. The holistic approach employed in this work plan provided a comprehensive framework for the effective and efficient achievement of selective sterilization. |
|
Sterilization device using nanomaterials |
|
The proposed device described in this study is a nanomaterial sterilization device that is intended for external decontamination purposes. This device is specifically designed for individuals who enter a room with dimensions that measure approximately 1 meter by 1 meter by 2 meters. The device employs a unique technology that utilizes a burst of steam that is infused with silver nanoparticles. The steam is directed towards the individual to eliminate harmful microorganisms, such as bacteria, viruses, and other pathogens that can be transmitted through the air or direct contact. The device under investigation for the present study follows a methodology that involves mixing silver nanoparticles stored in a reservoir with water and evaporating through ultrasonic evaporators while employing low-temperature and pressure conditions to produce a stable nanomaterial carried with cool steam for external distribution, allowing the silver nanoparticles to effectively eradicate pathogens present on the individual's skin, hair, or clothing. However, this device has a general external nanomaterial sterilization objective and does not have the selective ability to sterilize. The type and shape of the silver nanoparticles used can impact the effectiveness of the sterilization process. The sterilization process is initiated by an ultrasonic sensor, which detects changes in distance and transmits a signal to the controller. The controller subsequently emits two signals, one that changes the lighting color to indicate the start of the sterilization process and the other that activates the ultrasonic evaporators. The evaporators are firmly attached to the surface of tubes that are connected to the primary tank, where the mixture of water and silver nanoparticles is stored. Upon receipt of the signal from the controller, the evaporators generate steam saturated with silver nanoparticles that subsequently fall upon the skin and penetrate the clothing of the individual in question. While the effectiveness of the device has not been extensively tested due to some logistical challenges, this method remains the best theoretical approach for the practical use of silver nanoparticles in sterilization without affecting their composition or properties. Overall, this proposed device presents a unique and innovative approach to external decontamination, which has significant implications for public health and safety. |
|
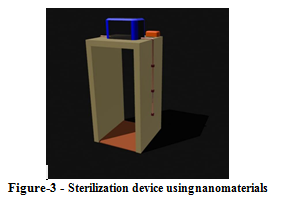 |
|
|
|
|
|
Selective sterilization by (Ag NPs) |
|
Antibacterial properties: |
|
The study found that the use of Ag NPs powder with a molecule length of 12 nm at concentrations of 50-60 μg cm-3 (10 5 CFU) and 20 μg cm−3 (104 CFU) resulted in an obvious inhibition of E. coli growth. Additionally, carbon-coated Ag NPs with a molecule length of 1-100 nm at a concentration of 75 μg ml−1 were found to be effective against V. cholera, P. aeruginosa, and Salmonella typhus, with P. aeruginosa and V. cholera exhibiting more resistance than E. coli and Salmonella typhus. Furthermore, the use of Ag NPs with a molecular length of 10-15 nm in an aqueous medium at a concentration of 25 μg ml−1 was effective against ampicillin-resistant E. coli, multi-drug-resistant Salmonella typhi, and S. aureus. The inhibitory effects of Ag NPs of various sizes and shapes on E. coli were also investigated, with truncated triangular molecules at a concentration of 1 μg, spherical molecules at 50-100 μg, and rod-shaped molecules at > 100 μg. Moreover, Ag NPs with a molecule length of 26 nm coated with Polymers/surfactants on standard strains and isolated from human clinical samples at concentrations of 1.69–13.5 μg ml−1 and 1 μg ml−1 SDS-modified Ag NPs were found to be effective against drug-resistant erythromycin-resistant E. coli O157:H7 and multidrug-resistant P. aeruginosa. The inhibitory effects of Ag NPs with a molecule length of 100 nm on drug-resistant and drug-susceptible bacteria were also investigated, with average concentrations of 79.4 nm and 71.5 nm for drug-resistant and drug-sensitive bacteria, respectively, for P. aeruginosa, Streptococcus sp., E. coli, and P. aeruginosa. Furthermore, the study found that the minimum growth inhibition was observed in Ag NPs with a molecule length of 13.4 nm on E. coli and S. aureus at concentrations > 3.3 nm (E. coli) and > 33 nm (S. aureus). The use of Ag NPs powder at a concentration of 100 μg ml−1 and Ag NPs coated with Oleate ions at ∼3 μg ml for both bacterial strains were also found to be effective against E. coli and S. aureus. Finally, Ag NPs coated with SDS with a molecule length of 8-50 nm were effective against E. coli, P. aeruginosa, and S. aureus at concentrations <7 ppm as shown in Table (1) [7]. |
|
Antifungal properties: |
|
Table(2) examined the effectiveness of using Ag NPs as an antifungal agent with a molecule length of approximately 3nm on 44 strains of 6 fungal species, including clinical isolates and ATCC strains of T. mentagrophyte and C. albicans, at IC 80: 1–7 μg ml−1. The results demonstrated complete growth inhibition. However, when Ag NPs coated with plastic catheters with a molecule length of 3–18 nm were used on the C. albicans strain, the growth of the catheter coated with Ag NPs (thickness 80-120 nm) was almost completely inhibited relative to C. albicans. Bare nanoparticles and 25nm sodium didosil sulfate anion (SDS) coated particles were also tested on Candida spp strain at 210 μg ml−1 for bare particles and 50 μg ml−1 for coated, showing a minimum level of inhibition of fungal growth. The same minimum level of growth inhibition was observed for C. albicans and Candida glabrata strains when treated with 5nm silver nanoparticles at a concentration of 0.4–3.3 μg ml−1, as well as for the T strain, Rubbrum, when treated with 28.2 – 100 nm at a concentration of 10 μg ml-1 [7]. |
|
Antiviral properties: |
|
Table (3), The efficacy of silver nanoparticles (Ag NPs) in inhibiting viral infections has been the subject of numerous studies. The effect of Ag NPs on various viruses, including HIV-1, HBV, RSV, monkeypox virus (MPV), MNV-1, and H1N1 influenza virus, has been investigated. When HIV-1 was treated with carbon-coated Ag NPs, polyvinylpyrrolidone (PVPs), and bovine albumin protein (BSAs), Ag NPs underwent a volume-dependent reaction with HIV-1 (1-10 nm), preventing the virus from binding to host cells. On the other hand, when HBV was treated with bare Ag NPs of different lengths (10, 50, and 800 nm), particles with a length of 10 nm were observed to inhibit viral replication. In the case of RSV, Ag NPs coated with PVP and BSA recombinant RSV fusion protein (F) inhibited RSV infection by 44%. Similarly, when MPV was treated with bare silver nanoparticles and polysaccharides with a molecule length of 10-80 nm, the molecules coated with polysaccharides at a length of 10 nm stopped the virus infection. Furthermore, when 11.2 nm molecule length Ag0 coated silver nanoparticles were used on the MNV-1 virus, the addition of 31.25 mg Ag0 m2 to the filter resulted in a 3.8 logarithm reduction for the virus compared to a decrease of 1.5 logarithms by the original filter. Finally, the H1N1 influenza virus showed active inhibitory activity when treated with Ag NPs at a molecule length of 10 nm. Overall, these findings suggest that Ag NPs can be a promising approach for antiviral therapy [7].
From this research, we can note that the smallest particle size, on average, affected viruses is 10 nanometers, and it affected most strains of viruses that were tested, except for the MNV-1 virus, which required a particle size of 11.2 nanometers [7]. Here we note that the smallest particle size of 10 nanometers can affect only viruses, almost without most fungi and other microbes [7]. We can take a look at the concept of selective sterilization from a comparator of the effect of silver nanoparticles at a concentration of 25 μg ml−1, where particles with a size of 10-80 nm without coating or with the coating (polysaccharide) were affected by the inhibition of MPV infection in the laboratory, but on the other hand, a concentration of 25 μg ml−1 for silver particles is the lowest inhibition concentration for fungi (C. albicans and Candida glabrata) If the size of the particle is approximately a nanometer and it is not coated with anything. In bacteria, we see this concentration with an inhibitory effect on growth, but in an aqueous medium exclusively, and the size of the molecule is between 10-15 nm without coating. Here we conclude that silver nanoparticles can target a specific microbe by discovering the appropriate formula for modifications to silver nanoparticles that have the greatest impact on this microbe [7].
|
|
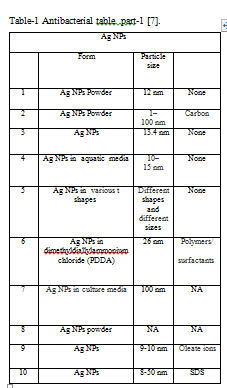 |
|
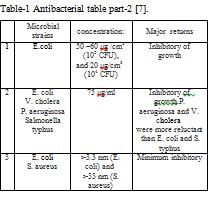 |
|
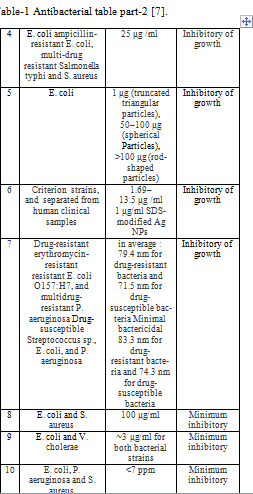 |
|
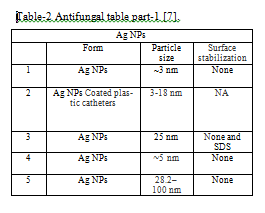 |
|
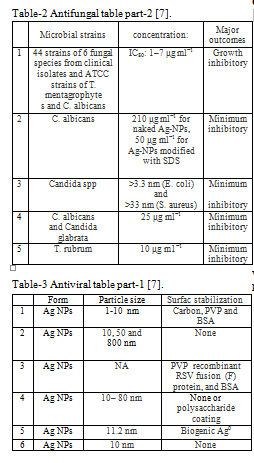 |
|
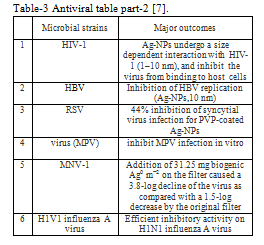 |
|
7-Conclusion |
|
In this study, the authors have examined the effects of silver nanoparticles on various microbes, which can be summarized by three major factors. Firstly, the nanoparticles within the size range of 1-10 nm have been found to disrupt essential cellular functions like permeability and respiration upon their adhesion to the outer surface of a bacterial cell [4]. Secondly, these nanoparticles have demonstrated the ability to penetrate the bacterial cell wall and react with sulfur and phosphorus-containing DNA [4]. Lastly, the release of Ag+ silver ions as a product of these reactions has exhibited anticonvulsant effects and has enhanced the effectiveness of silver nanoparticles against bacteria [4]. However, it should be noted that there can be different effects on different strains of microbes, such as bacteria, fungi, and viruses. Hence, selective sterilization can be achieved through cross-referencing experimental results with a literary review of the effects of silver nanoparticles on microbes. Our findings suggest that it is possible to allocate sterile material with a specific strain, such as targeting virus strains by using Ag Nps with a molecule size of 10 nanometers [7]. In this research, the authors have discussed the same concentration of sterile material having different effects on different strains through specific modifications on the nano-sterile material. The authors suggest that further experiments and tests on the modification of Ag Nps can lead to the development of an ideal formula that enables the total annihilation of a specific strain |
|
8-References: |
|
|
|
1. Argitos nanosilver, Available: Differences between silver nanoparticles and silver ions - Наносеребро Аргитос (argitos.com) |
|
2. Anna K., Mateusz S., Eva K., Jacek R., Anna Ł., Gabriela B.P. (2018). Similarities and Differences between Silver Ions and Silver in Nanoforms as Antibacterial Agents. International Journal of Molecular Sciences, 19(2), pp.2. |
|
3. Ergi T., Mevlüt A., Berrak G.B., Zeynep P.Ç. (2022). Microbial silver resistance mechanisms: recent developments. World Journal of Microbiology and Biotechnology, 38(9), pp.2-4. |
|
4. Yapeng Z., Xuanhe P., Shijing L., Congyuan J., Linqian W., Yurong T., Guojun W., Gan D., |
|
Liyu C. (2020). Quantitative Proteomics Reveals the Mechanism of Silver Nanoparticles against Multidrug-Resistant Pseudomonas aeruginosa Biofilms. Journal of Proteome Research, 19(8), pp. 3109–3122. |
|
5. Tamara B., Francisca M.B., Paul J., Nelson C. (2021). Silver Nanoparticles and Their Antibacterial Applications. International Journal of Molecular Sciences, 22(13), pp.1-8. |
|
6. Qing Y., Cheng L., Li R., Liu G., Zhang Y., Tang X., Wang J., Liu H., Qin Y. (2018). Potential antibacterial mechanism of silver nanoparticles and the optimization of orthopedic implants by advanced modification technologies. Natural Library of Medicine, 5(13), pp. 3317-3327. |
|
7. Quang H.T., Van Q.N., Anh T.L. (2013). Silver nanoparticles: synthesis, properties, toxicology, applications and perspectives. Advances in Natural Sciences: Nanoscience and Nanotechnology, 4(3), pp. 5-10. |
|
8. Niloofar J., Fatemeh M. (2022). Nanomaterial-Augmented Formulation of Disinfectants and Antiseptics in Controlling SARS CoV-2. Natural Library of Medicine, 14(2), pp. 105-119. |
|
9. - Sundararaj S., Kei M., Takeshi M., Yutaro Y., Akihide R. (2020). Potent antiviral effect of silver nanoparticles on SARS-CoV-2. Natural Library of Medicine, 533(1), pp. 195-200. |
|
10. Roberto V.M., Jose L.R. (2020). Nanotechnology as an Alternative to Reduce the Spread of COVID-19. preprints.org, 14(21), pp. 2-7. |
|
11. Maaz Kh. (2018). Silver Nanoparticles - Fabrication, Characterization and Applications. IntechOpen. P. 288. |
|
12. Quang H.T., Van Q.N., Anh-Tuan L. (2013). Silver nanoparticles: synthesis, properties, toxicology, applications and perspectives. Vietnam Academy of Science & Technology, 4(3), pp.6-8. |
|
13. Mitra K., Elham Gh., Shahla K., Zahra H., Afshin M.B. (2015). Effects of silver nanoparticles on human health. European Journal of Nanomedicine, 7(1), pp. 57. |
|

|
|
|